Increase
Accessibility to Healthcare
CelluCov aims to provide sustainable solutions that are accessible to everyone, without sacrificing quality or performance of the device. While eco-friendlier manufacturing processes are often more expensive, we make a conscious effort to utilize materials that maintain our product’s affordability at or below the cost of traditional tests on the market.
Maintain
Quality of Testing
Our team at CelluCov is continuously exploring new and innovative ways to create eco-friendlier lateral flow assays. One of the latest initiatives we are working on involves testing the ability of gold nanoparticles and functionalized quantum carbon dots to cellulose-based materials to create a fully functional and biodegradable COVID lateral flow assay device.
Reduce
Medical Waste
Since the rise of the pandemic, the amount of medical waste specifically from testing worldwide has increased, generating 2,600 tons of non-infectious waste and 731,000 liters of chemical waste. This increase is damaging our environment and created a need for a more eco-friendly method of testing — biodegradable lateral flow assay based testing kits.
Table of Contents
- Team Members
- Abstract
- Problem Statement
- Rationale
- Prototype
- Material Properties and Degradation Testing
- Limitation
- Future
- Acknowledgements
- References
Team Members
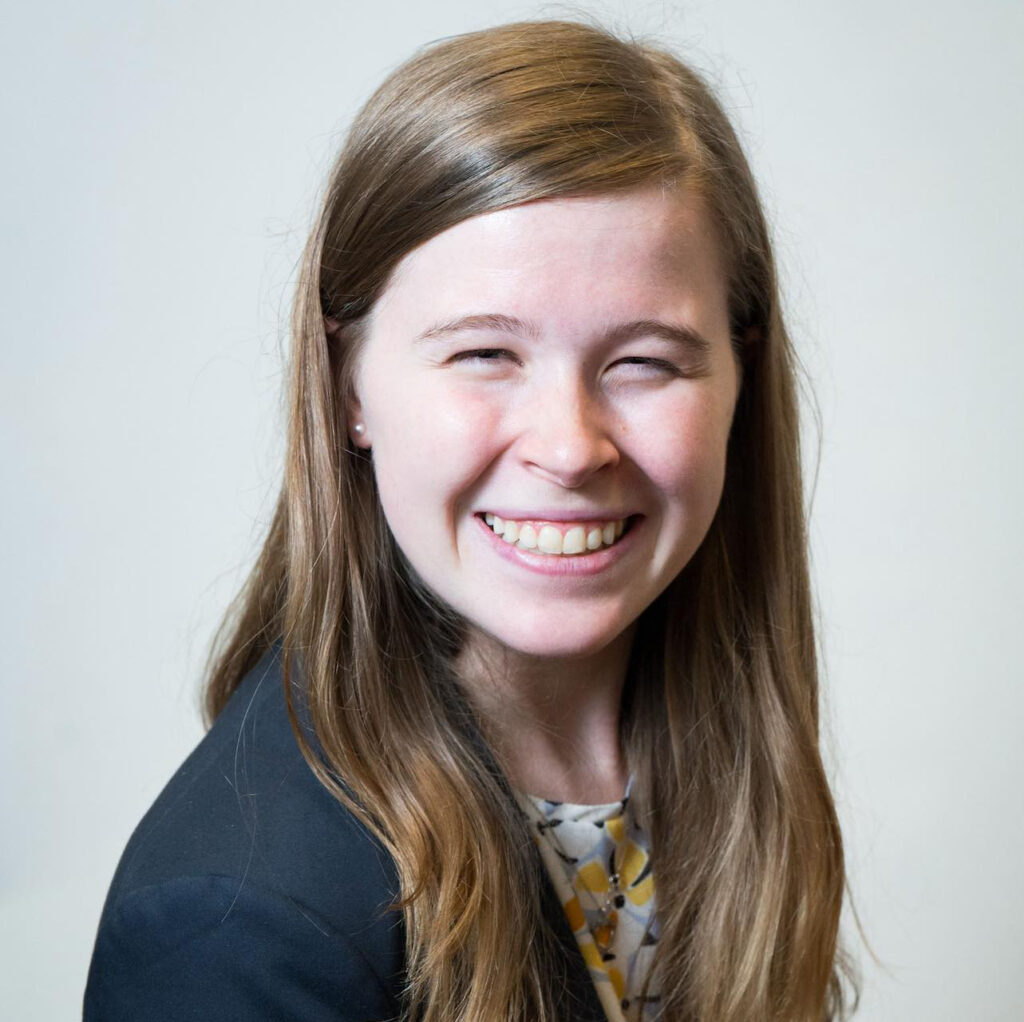
Katherine Broun (she/her)
Incoming Ph.D. Student in Biomedical Engineering at Duke University
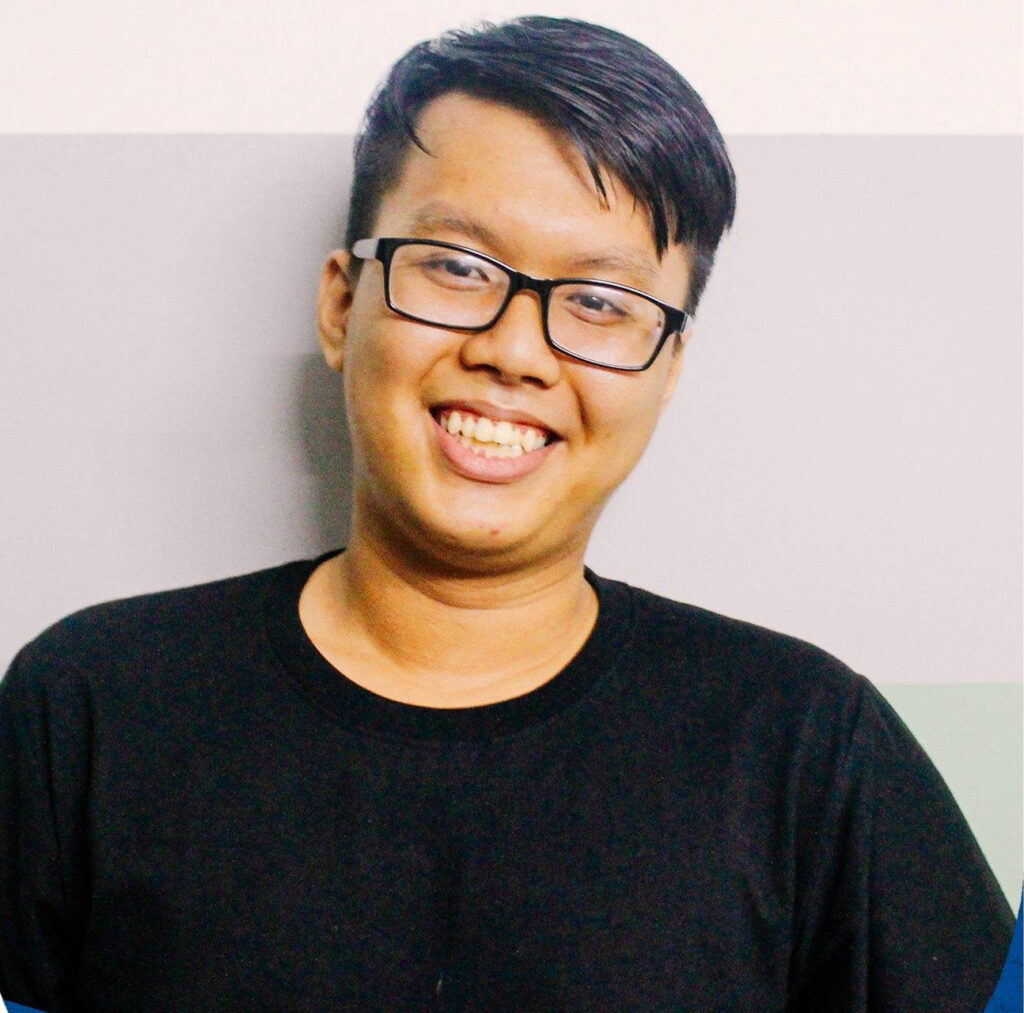
Duy Doan (he/him)
Incoming M.S. Student in Biomedical Engineering at University of Rochester – CMTI
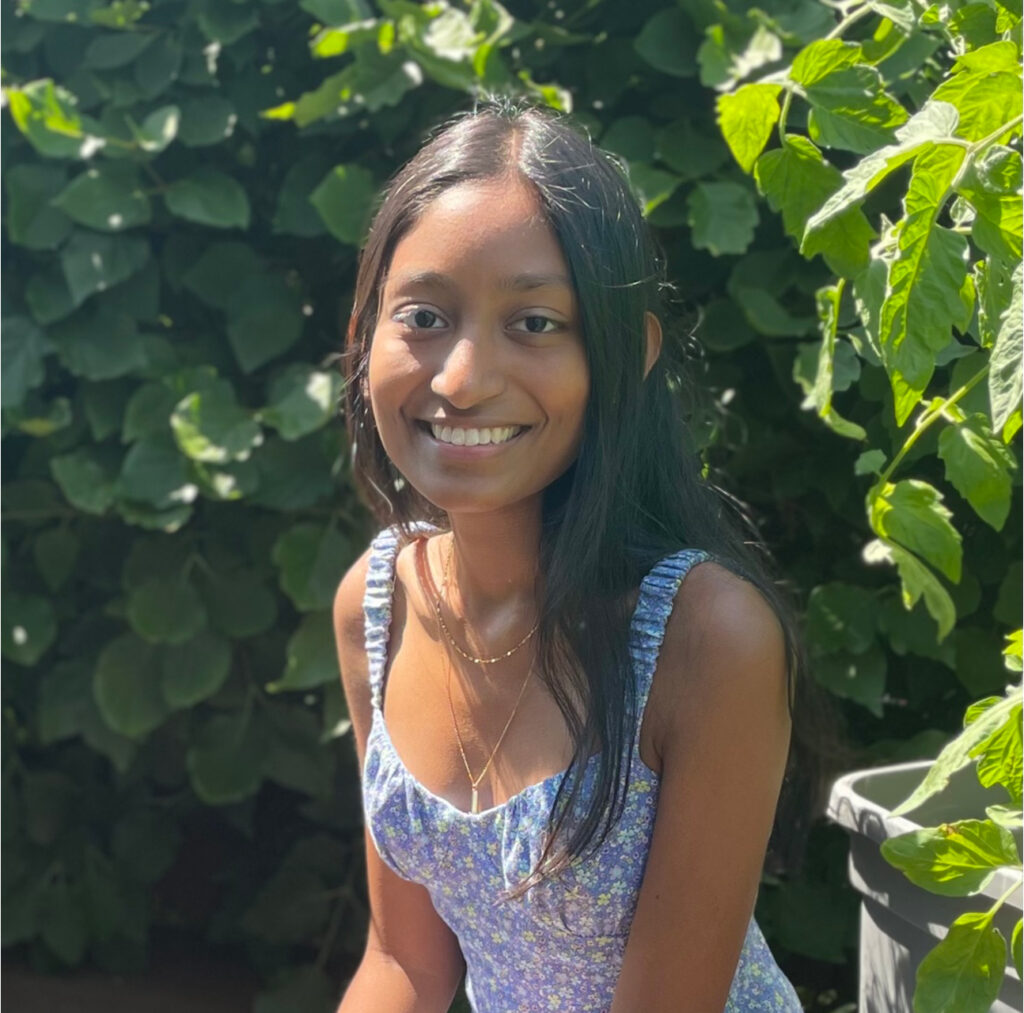
Judy Monickaraj (she/her)
Incoming M.S. Student in Biomedical Engineering at University of Rochester – CMTI
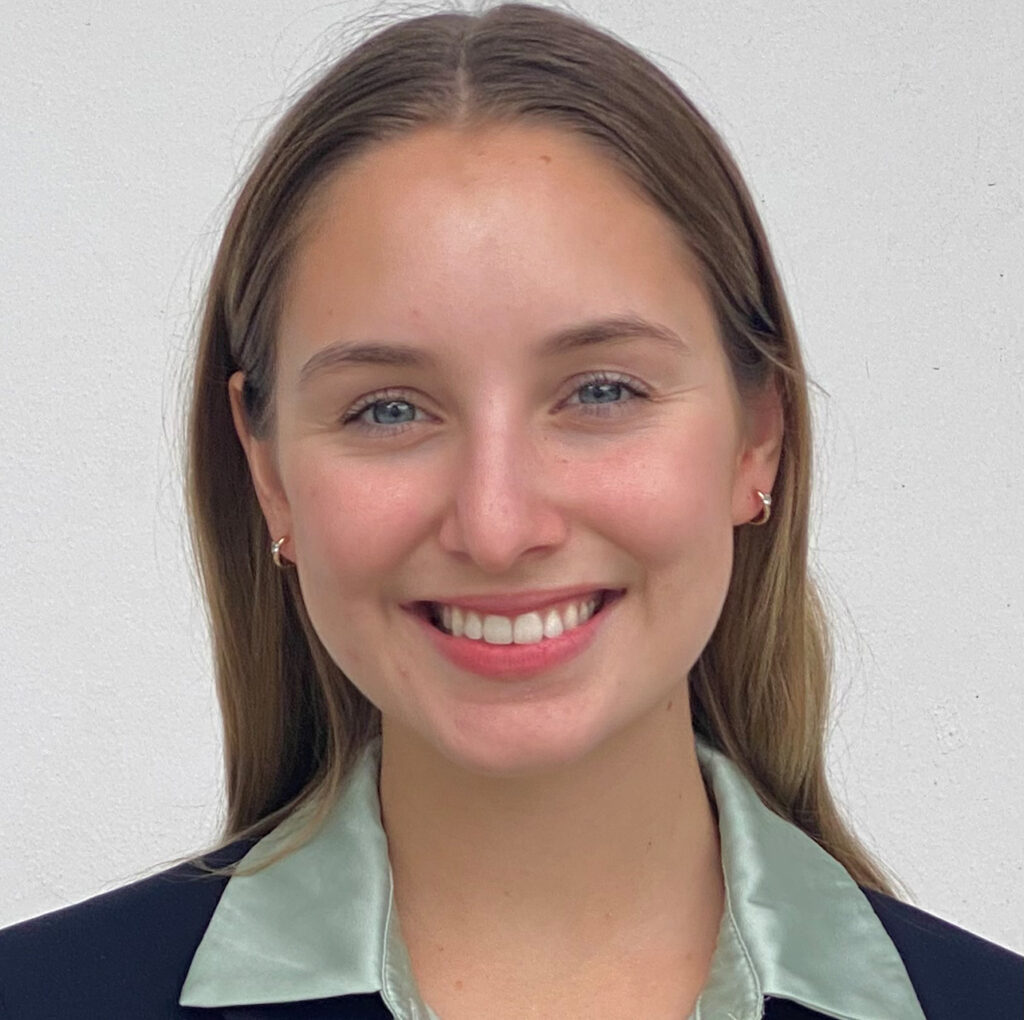
Claudia Garcia (she/her)
Incoming Ph.D. Student in Biomedical Engineering at Tulane University
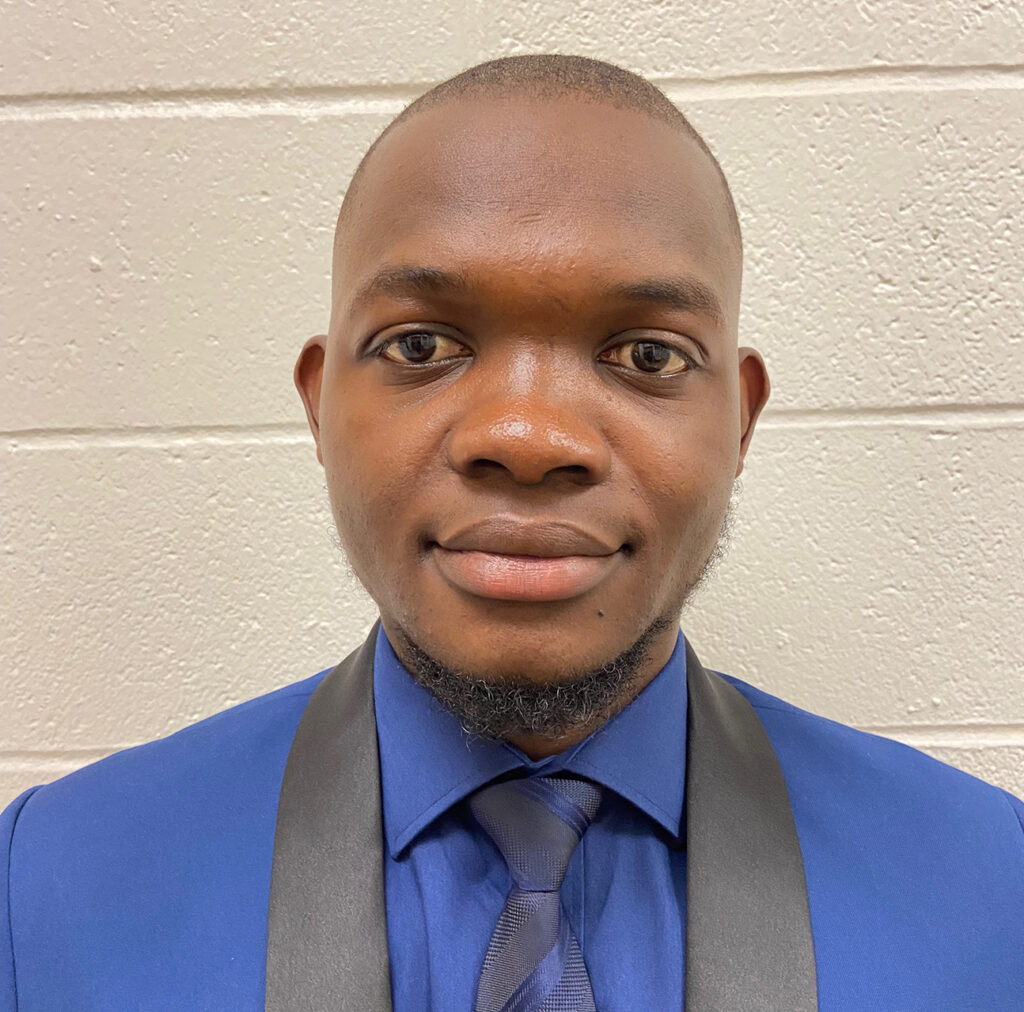
Miguel Yakouma (he/him)
Incoming M.S. Student in Biomedical Engineering at University of Rochester – CMTI
Abstract
Since 2020, the amount of medical waste from COVID testing worldwide has increased, generating 2,600 tons of non-infectious waste. This increase is damaging our environment and created a need for a more eco-friendly method of testing — biodegradable lateral flow assay based testing kits. Our goal is to create a COVID lateral flow assay which can degrade in soil or ground sewage water lines. Our team will create a device which can degrade naturally in six months to one year, be manufacturable at 10 cents per device, user friendly, and look similar to previous designs on the market, given the current constraints of creating a device which will be well received in lower-middle income countries (LMIC), including Kenya. To facilitate degradation, our team has discovered two enzymatic pathways which catalyze the initial degradation reaction of our chosen plastic material, PHB, for the major test kit components. When thinking about moisture in LMICs, we included a desiccant in our packaging to ensure no moisture would invade our device prior to use. Given the environment, creating a low-cost design is vital; however, given the biodegradability constraints which require more expensive materials, one may be at odds with the other. Since our main focus is biodegradability, our team is currently creating a proof of concept test; however, with additional work, we will utilize carbon quantum dot nanotechnology to create test lines in our lateral flow assay which can degrade simultaneously with other test components. Through our design process, we have begun prototyping all test components. Through this process, we have discovered limitations with 3D printing and other fabrication mechanisms as well as the chemical processes of proof of concept testing. With our future work, we will confirm the degradation ability as well as the sensitivity of our created lateral flow antigen test.
Problem Statement
While current lateral flow assay antigen tests are highly sensitive and strong for disease detection purposes, they are made with materials that are not environmentally friendly. Our goal as a team is to create a COVID lateral flow assay antigen test that can degrade in a non-toxic manner in soil or ground sewage water lines while still maintaining low cost per unit and high sensitivity levels.
Rationale
When designing our device, we came up some needs and wants for our final product based on our customer’s intent of distributing the devices to low-income communities with poor waste management techniques. We prioritized degradation within 6 months, all products and degradation byproducts having a toxicity of less than 10 ppm, and a familiar design to aid in user friendliness and combatting medical mistrust. Some of our wants include having a shelf-life of about 3 years (similar to current technology), a cost of about 10 cents per unit, and producing a test result within 15 minutes.
Rank | Need | Goal | Want | Goal |
1 | Fast Degradation Time | ≤ 6 months | Long Shelf Life | 1.5 years |
2 | Non-Toxic | ≤ 10 ppm toxicity of byproducts | Low Cost of Production | ~ $ 1.00 / device |
3 | Familiar Appearance | Similar appearance to rapid tests on the market | Fast-to-Use | 10-15 minutes |
Prototype
We proposed to keep the current design of a COVID test while replacing the materials with biodegradable ones. For the test strip, the sample and absorbent pads are made from wood pulp sponges. The membrane pad uses cellulose-based materials that allows lfa and antibodies to adhere. Encasing the test strip is the PHB cassette and PVA bags are used in place of mylar. The buffer vial uses PHA for the body and PHB for the cap. The nasal swab is cotton tip swab with cellulose stick. The desiccant is replaced with nano charcoal in paper bags and the instruction will be printed using algae ink on water soluble papers.
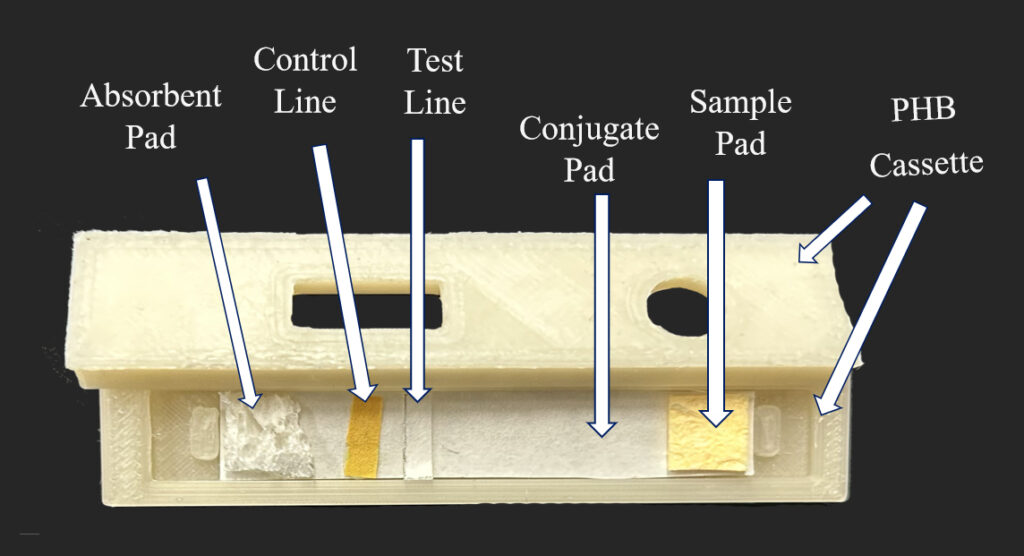
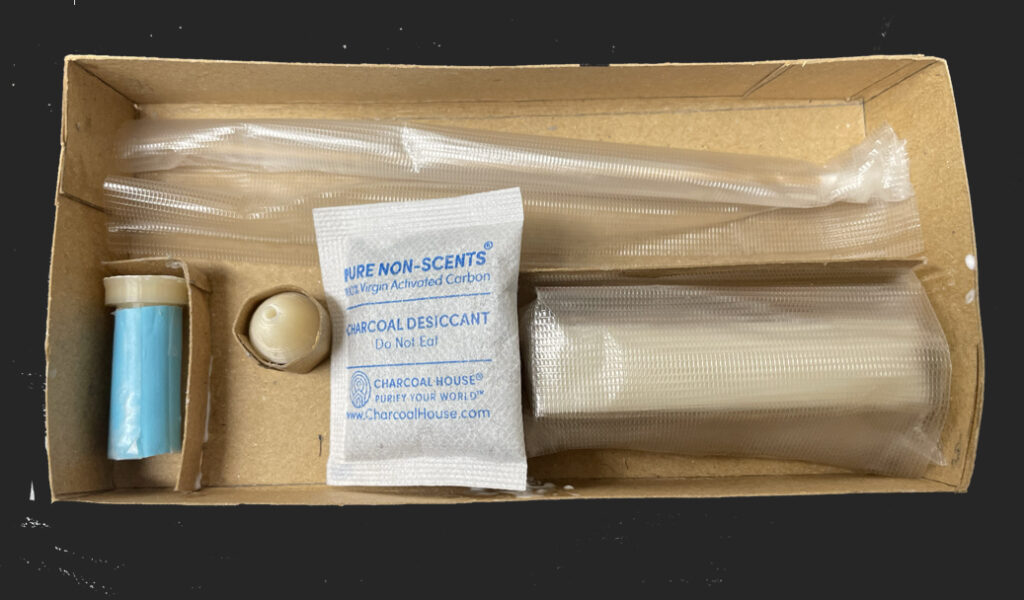
Materials Properties and Degradation Testing
Our design process began with the hydrophilicity testing of the new biodegradable materials, cellulose wood pulp sponges, to ensure that their functions or properties are not very different from the current sample and absorbent pads. After measuring the contact angle of several cellulose wood pulp sponges, we were able to finalize material selection that resembles the sample and absorbent pads in current COVID tests.
COVID test devices rely on lateral flow assay (LFA) technology to detect COVID antibodies so it is necessary to ensure the new materials of the test strip can function properly as a LFA device. The test is a proof of concept test designed to mimic the negative and positive result of a COVID test in real life.
To determine whether our materials of choice can biodegrade within the 6 months to 1 year time span, we set up degradation tests in various conditions to measure the dissolved O2 concentration, mass loss, and surface roughness using confocal images of the PHB discs.
Hydrophilicity Test
The team used an optical tensiometer from the Chemical Engineering department to make measurements of the contact angles between the water droplet and the materials at moment of contact. We measured the contact angles of the sample and absorbent pads used in current COVID test device and those of the novel materials in the test strip. Statistical analysis were performed on the contact angles of existing and proposed materials to ensure the proper function of the test strip. We anticipate performing a two sample t-test to evaluate the difference in hydrophilicity.
The sessile drop method is a good measurement to quantify the hydrophilicity of a material as it measures how water interacts with the material. Since contact angle is proportional to the hydrophobicity of the materials, the bigger the contact angle, the more hydrophobic the material. It is determined that sponge 1 has similar hydrophilicity to the existing absorbent pad and sponge 3 has similar hydrophilicity to the existing sample pad.
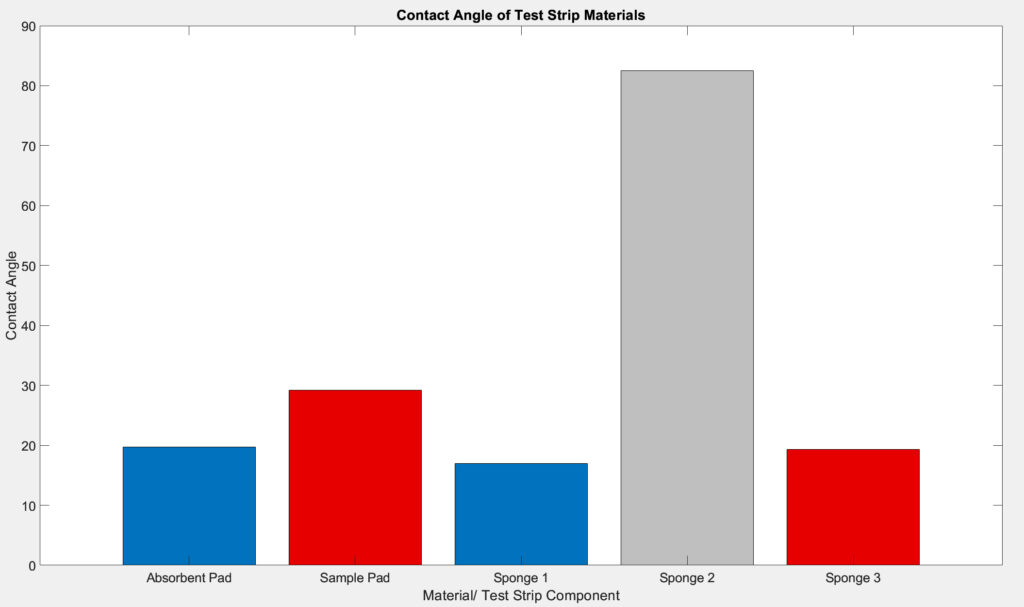
LFA Proof-of-concept Test
This test is a proof of concept test designed to mimic the negative and positive results of a COVID test in real life. In either case, the control line must show visual indications that the testing solution carries conjugates of COVID antigen as a control while the testing line must show visual indication only in the positive result case. The test was performed at normal room temperature of 20oC and atmospheric pressure of 1 atm. For both cases, the testing solution was made of luminol. In the positive result case, diluted hydrogen peroxide (<3%) was added to the testing solution to mimic nasal fluid from COVID patients. The control line is made of litmus paper and is expected to show blue color indication as it detects basic luminol solution in both scenarios. The testing line is made of cellulose paper with traces of diluted FeSO4 solution to represent COVID antibodies on the test line. In the negative result scenario, the test line remained white whereas in the positive result scenario, it became red. The difference in color of the control line (blue) and test line (red) is to ensure that customers do not misinterpret the test results, considering that the test is an over-the-counter device and will be used without the presence of medical professionals.
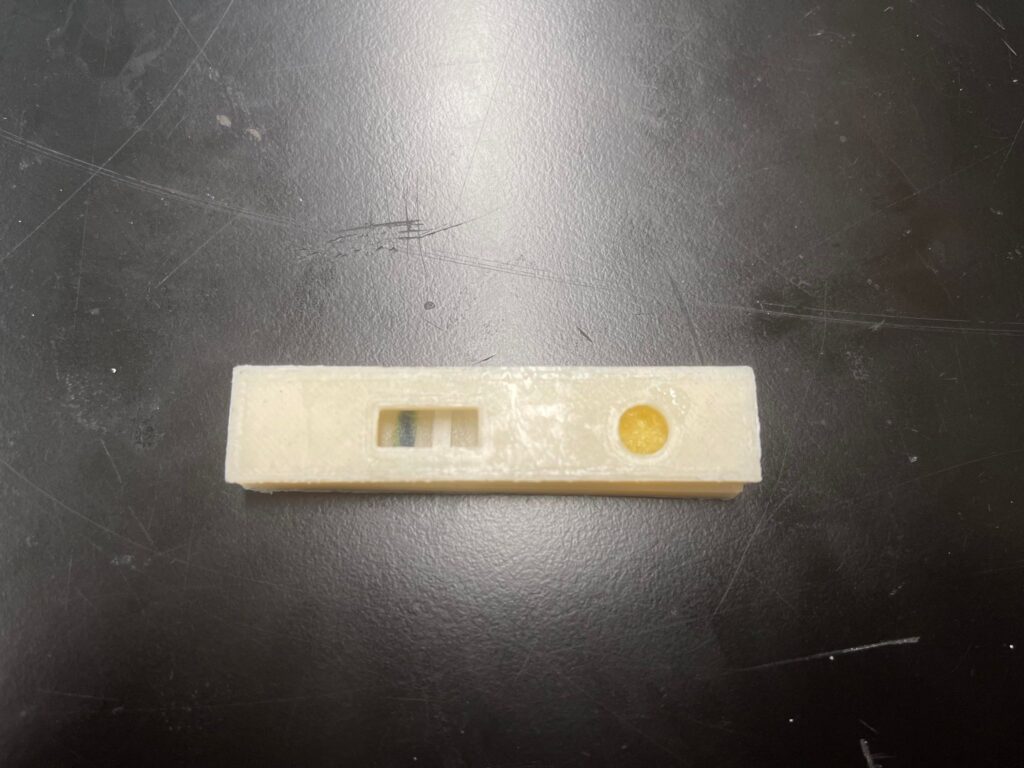
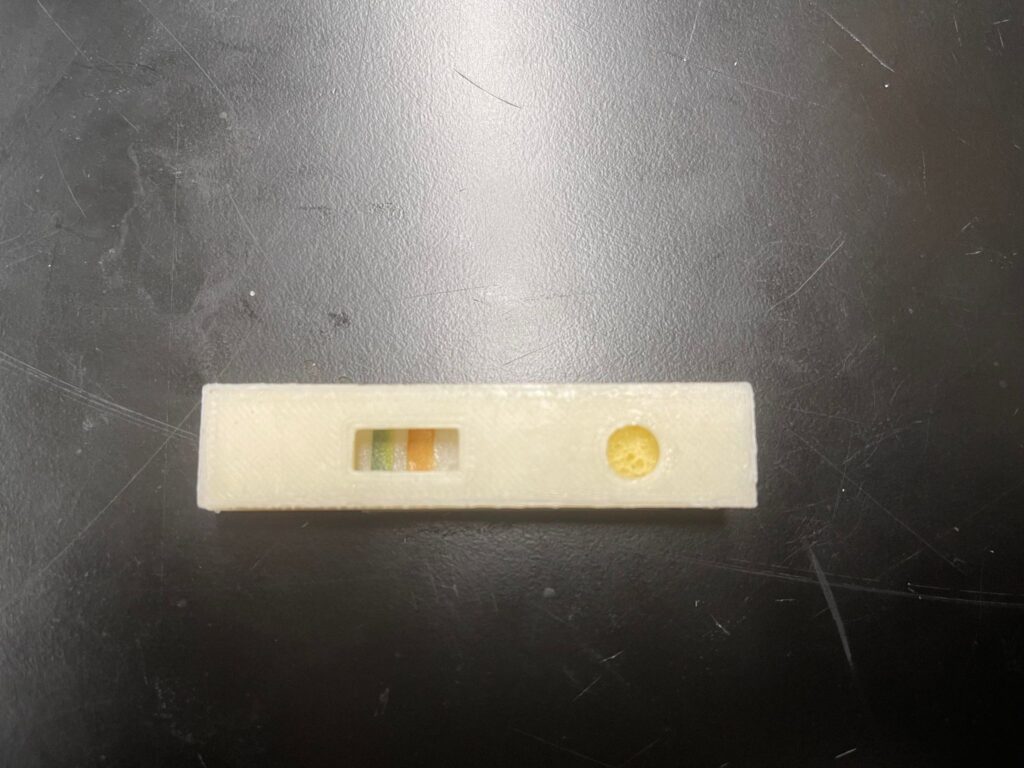
Mechanical Test
One half of the PHB cassette underwent tensile testing, in which force was continually added to the cassette to determine the point at which the material began to weaken (ultimate strength). We found this value to be lower than other plastics, indicating our material is more brittle and susceptible to degradation by natural mechanical forces.
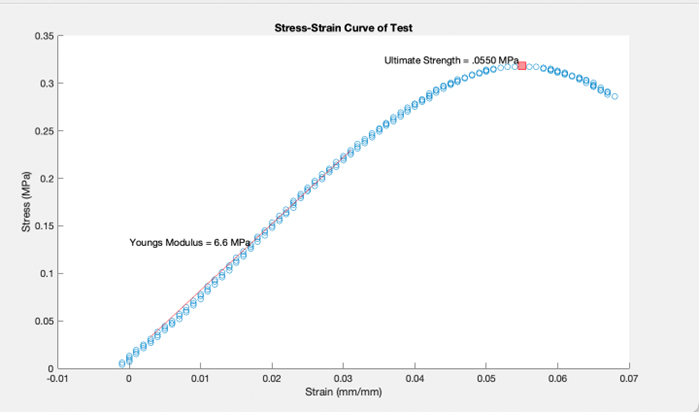
Dissolved O2 Concentration Test (ASTM D5511)
A PHB disc was placed in artificial seawater based on the ASTM standard D6691–17 recipe, along with septic bacteria. A hole was created in the container lid for the Vernier Oxygen Sensor Probe to be placed into, and this hole was sealed with parafilm to create anaerobic conditions. Over the course of a week, the dissolved O2 concentration decreased by 1 mg/L, suggesting the microbes are degrading the PHB for nutrients to stay alive and consuming the dissolved O2.
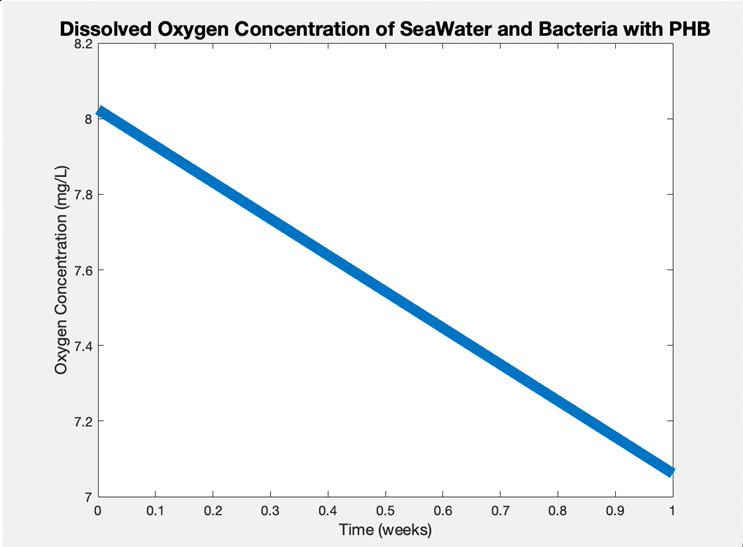
Mass Loss Test
For the mass loss test of our device, we performed a test similar to that of a soil burial test. It was carried out at room temperature (~20°C) and samples were covered with aluminum to protect them from UV light to simulate the conditions of a septic tank. The test was performed in triplicate per condition which include (A) a container with just PHB disc and the buffer solution, (B) a container with PHB disc, buffer solution, and distilled water, (C) a container with PHB disc, buffer solution, and water from the Genesee River, (D) a container with PHB disc, buffer solution, and sewage bacteria, (E) a container with PHB disc, buffer solution, distilled water, and sewage bacteria, and (F) a container with PHB disc, buffer solution, water from the Genesee River, and sewage bacteria. Containers that include the sewage bacteria was prepared by adding sewage bacteria under and on top of the PHB sample. The PHB discs were 3D printed and the sewage bacteria were obtained from septic tank treatment that contains live bacteria. The PHB discs were removed and weighed at intervals of 2 days for a timeline of 3-4 weeks to view both long term and short term degradation. The PHB discs were then be brushed softly, washed with distilled water several times, and dried with kimwipes.
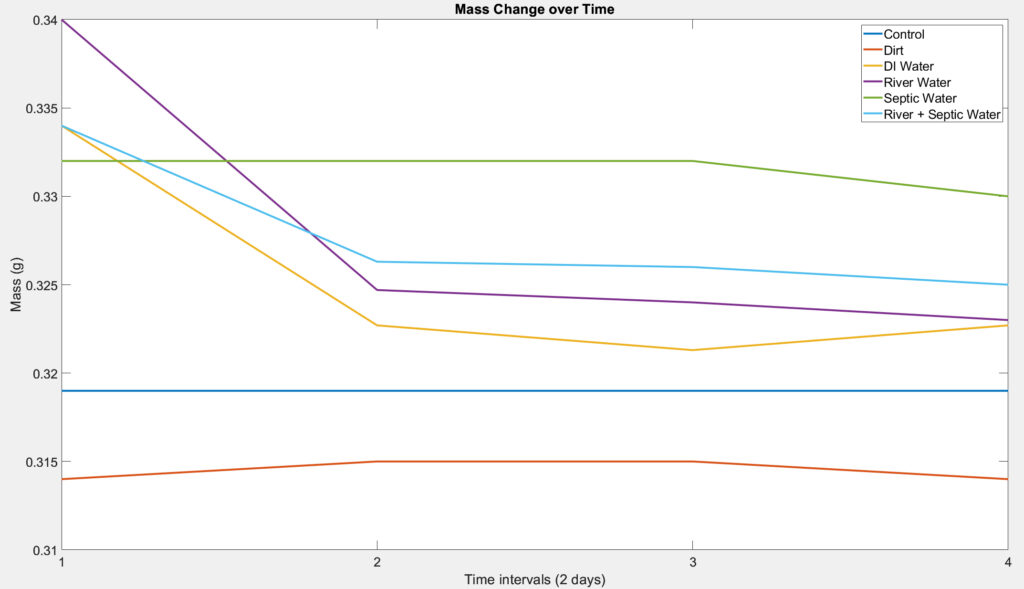
Confocal Images of PHB Surfaces
To quantify surface level changes in degradation, confocal imaging technology was utilized. Our team took images with magnification of 20x head on of the surface and several viewing angles in the lattice structure to be able to differentiate the breakdown of PHB. Additionally, we took images on a tilted surface stage to be able to see notches of degradation at the micron scale. The size of these notches as well as the different in surface roughness were quantified in post process image analysis in MATLAB in order to determine the amount of materials which was removed from the sample. The PHB discs were made of both circular and rectangular shapes in order to differentiate degradation between the surfaces and corners.
Through the confocal images we determined that the average surface roughness of PHB in control setting is 3.4 μm and in septic + river setting is 7.6 μm. In other words, the surface roughness of PHB in septic + river setting differs to the control setting by the amount of two times the standard deviation, meaning there is a statistical difference between the surface roughness of two samples. It could be concluded that PHB degrades in settings containing septic bacteria, which further reduces the overall degradation time.
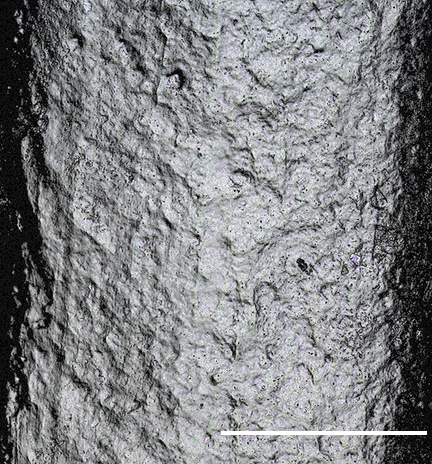
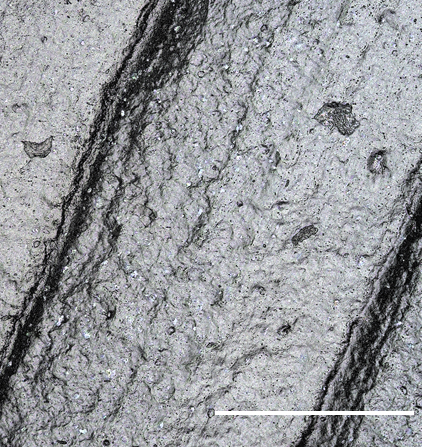
Limitation
It comes to our knowledge that we have limited time for the testing phase and more data needs to be collected before the precise degradation time is determined. Cost is also a problem since our goal was $1.00 per device and the estimated current cost of production is about $1.5 per device without distribution and regulatory costs.
The preservative currently used in testing buffer solution of COVID test, sodium azide (NaN3), does not degrade and is extremely toxic for human at concentration above 133 ppm. However, we believe the amount of NaN3 in 0.3 mL of buffer solution is unlikely to be more toxic than most substances in the septic system. Temperature is also another concern as COVID devices should be stored in temperature ranging from 39 – 86 degrees Fahrenheit while Conex shipping containers can reach 135 degrees Fahrenheit (57 degrees Celsius).
Future
Testing Plans
As we have seen signs of degradation, we would like to continue the O2 concentration measurements and mass loss degradation tests to have a better understanding of the total degradation time. We would also like to test the ability to adhere of gold nanoparticles and antibodies on cellulose-based materials to transit from a proof-of-concept device to a functional biodegradable COVID test.
Manufacturing Considerations
We would like to expand our market by adapting the device to be able to flush down U.S. septic systems, such as the LIA flushable pregnancy test that is currently on the market. We are looking into functionalizing the biodegradable quantum carbon dots to replace current non-degradable gold nanoparticles in antibody tagging as a mean to reduce manufacturing costs. Moreover, we are working on the injection molder capability of the PHB cassette and buffer vial to reach our goal of low production cost.
Acknowledgements
We would like to express our deepest appreciation to our customers Jeff Beard and Dr. Benjamin Miller for providing us the opportunity to work on an amazing project and assisting us during the testing phase of the project. We are extremely grateful to our supervisor Dr. Richard Waugh for guiding us throughout the project, providing us with constructive criticism, and pushing us to deliver our best. We would like to thank Dr. Anne Meyer for sharing her insights about biodegradable materials and providing feedback to our testing plan. Thank you to Martin Gira for providing the team with tools, equipment, and materials throughout the course of the project as well as your knowledge of 3D printing processes. We would like to extend our thanks and appreciation to Dr. Scott Seidman, Dr. Kanika Vats, Taimi Marple, and Mahllet Beyene for meeting with us throughout the past two semesters and providing us with helpful resources. Last but not least, we extend our gratitude to our colleagues who have contributed in various ways to make this project successful. Their valuable inputs and support have been invaluable to us.