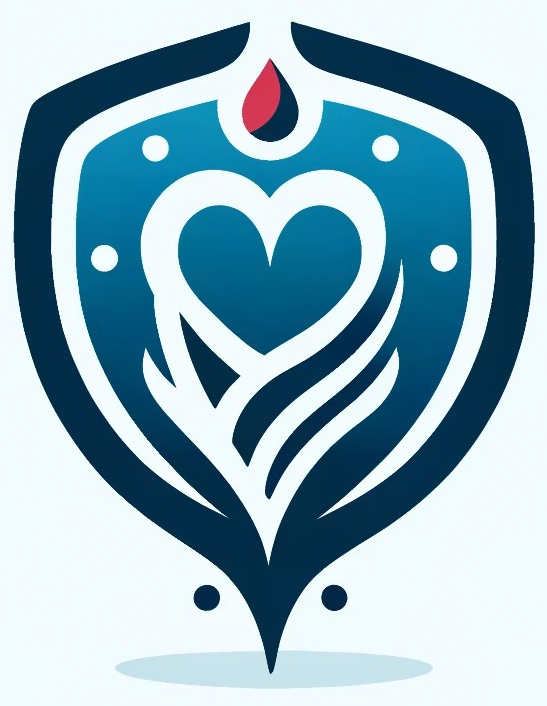
Table of Contents
- Team Members
- Background
- Problem
- BioDesign Statement
- Rationale
- Requirements
- Prototype
- Testing
- Future
- Acknowledgement
- References
Team Members
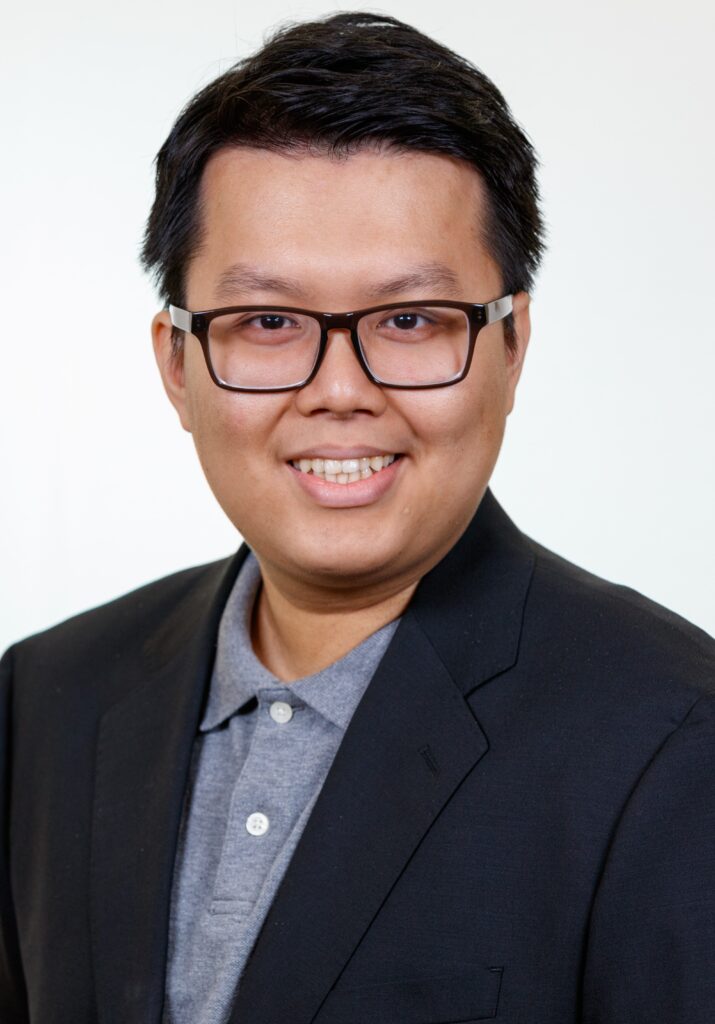
Duy Doan
M.S. Student in Biomedical Engineering at University of Rochester – CMTI
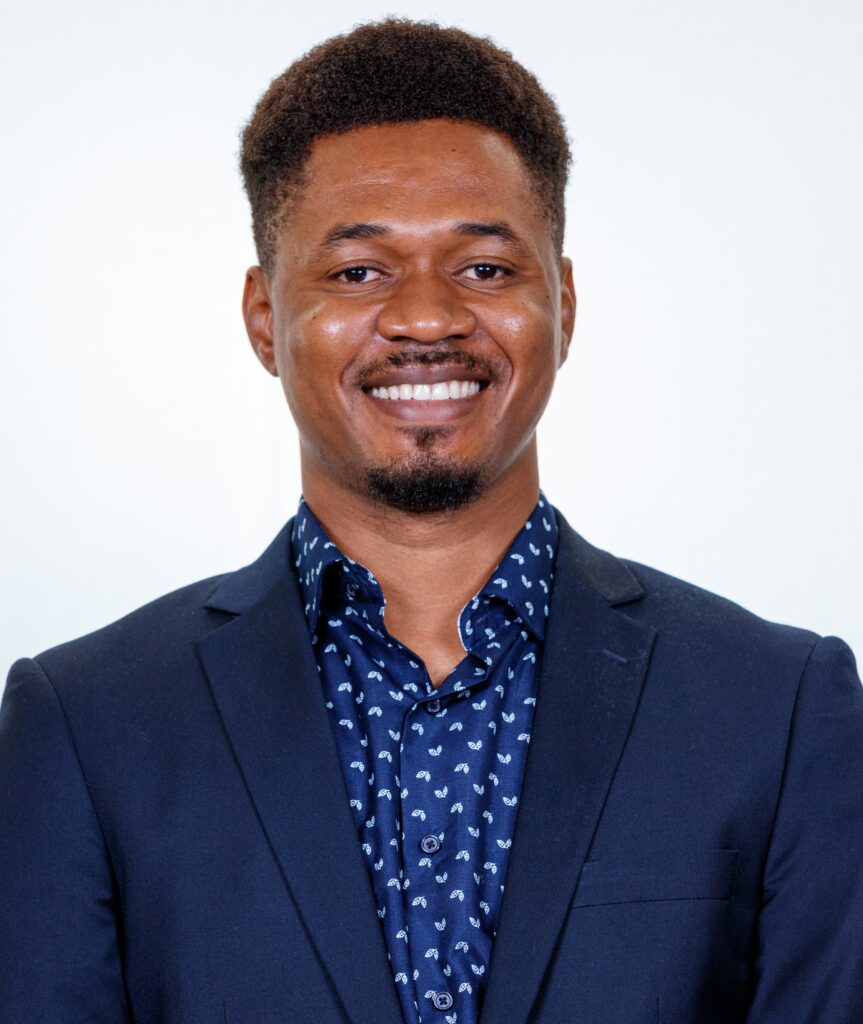
Ben Amadi
M.S. Student in Biomedical Engineering at University of Rochester – CMTI
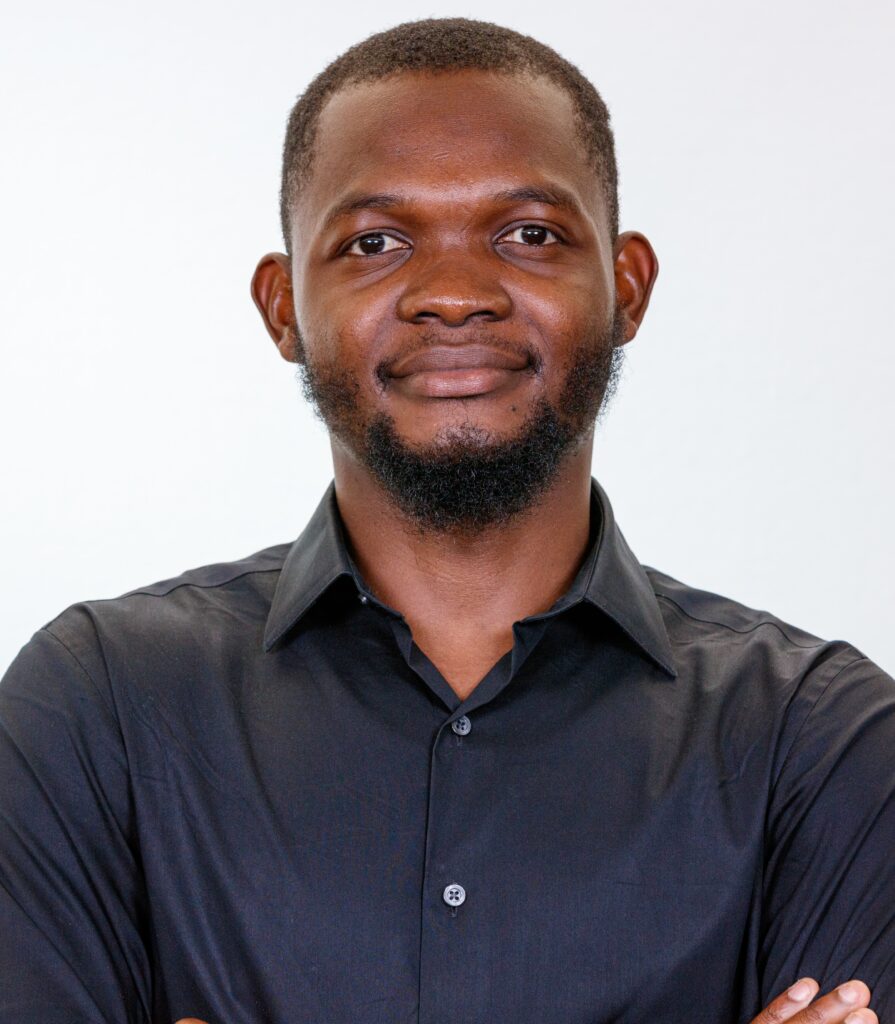
Miguel Yakouma
M.S. Student in Biomedical Engineering at University of Rochester – CMTI
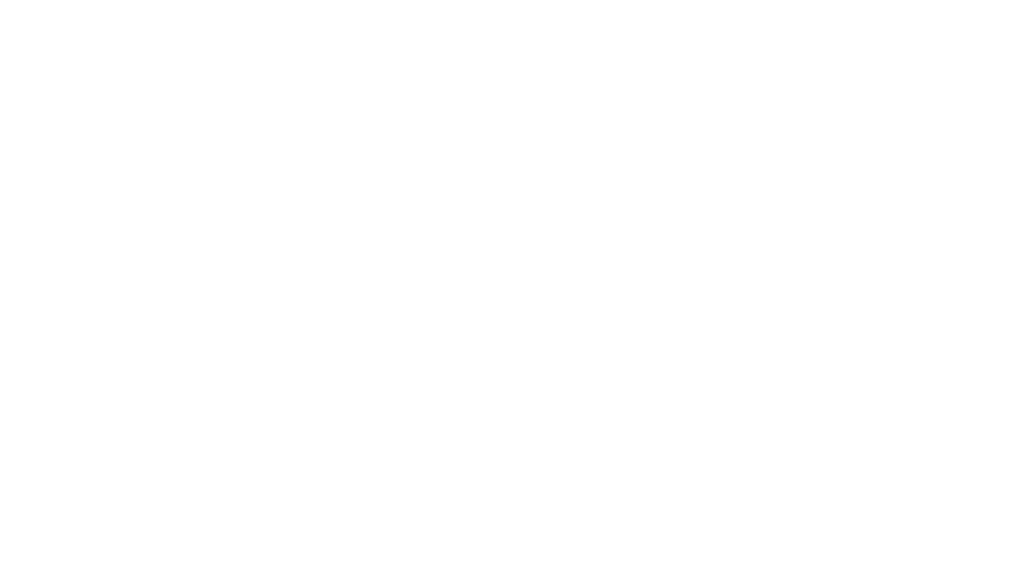
empty
empty
empty
empty
Background
Approximately 6.7 million Americans aged 20 and older suffer from heart failure, with projections estimating an increase to 8.5 million by 2030 [1]. The prevalence of heart failure in the overall population ranges from 1.9% to 2.6% and is expected to rise to 8.5% among 65–70-year-olds [1].
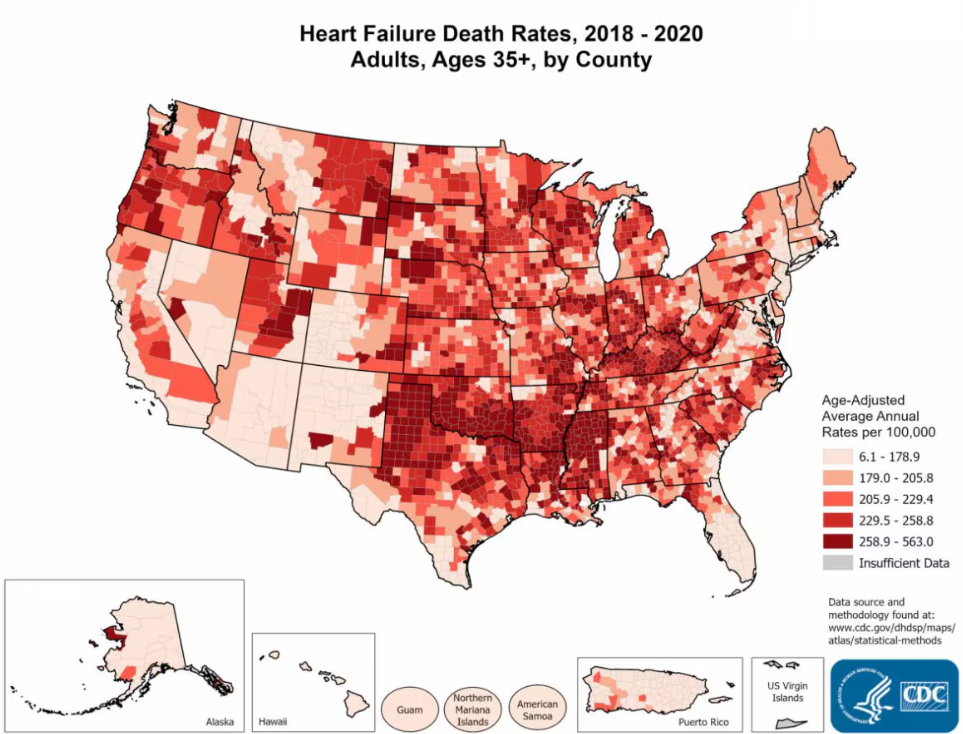
Congestive Heart Failure (CHF) is a condition where the heart’s ability to pump blood is less efficient than normal, which can lead to fluid buildup in the lungs and other parts of the body. This condition is often progressive and can be the result of various underlying heart problems. The heart, a muscular organ tasked with circulating blood throughout the body, faces challenges in CHF. In some cases, the heart’s muscles weaken, making it less effective at pumping blood (systolic dysfunction). In others, the muscles stiffen, hindering the heart’s ability to fill with blood (diastolic dysfunction). These issues lead to reduced blood and oxygen flow to the body’s tissues.
As the heart labors under these conditions, the body tries to compensate. It might activate the sympathetic nervous system and hormonal pathways, initially helpful but eventually worsening the heart’s condition. The heart might also undergo remodeling, changing in size and shape, which can further impair its function. The symptoms of CHF are as varied as they are distressing. People with CHF often experience shortness of breath, particularly during physical activity or lying down. They might notice swelling in their legs, ankles, and feet due to fluid accumulation. Fatigue and weakness are common, making everyday activities challenging. Some might have rapid or irregular heartbeats, a persistent cough, or sudden weight gain due to fluid retention [2].
Treating CHF is a multifaceted endeavor. It often starts with lifestyle changes like adopting a low-sodium diet, managing fluid intake, and engaging in appropriate exercise. Medications play a crucial role, too. These might include angiotensin-converting enzyme inhibitors and Angiotensin II Receptor Blockers to lower blood pressure, beta-blockers to slow the heart rate, diuretics to reduce fluid buildup, and others to strengthen heart contractions or balance electrolytes. In more advanced cases, medical devices or surgery might be necessary. Pacemakers can help regulate the heart’s rhythm, while implantable cardioverter defibrillators can prevent life-threatening arrhythmias. Some patients might require coronary bypass surgery, heart valve surgery, or even the implantation of ventricular assist devices. In the most severe cases, a heart transplant might be the only viable option.
Patients experiencing severe heart failure who are ineligible for transplantation or are on the transplantation list may qualify for the placement of a supportive mechanical pump during Left Ventricular Assist Device (LVAD) surgery. In this surgical procedure, cardiologists undertake coring at the apex of the left ventricle to facilitate the insertion of the HeartMate 3 (HM3) device, utilized as a destination therapy (DT) due to its enhanced durability spanning five to ten years [3]. The HM3 consists of two primary components: the apical cuff, securing the pump with sutures at the left ventricular apex, designed for straightforward surgical placement and a low risk of hemolysis [3]. Hemolysis is when red blood cells break down more rapidly than usual, and thrombosis refers to a blood clot within blood vessels that limits blood flow.
Problem
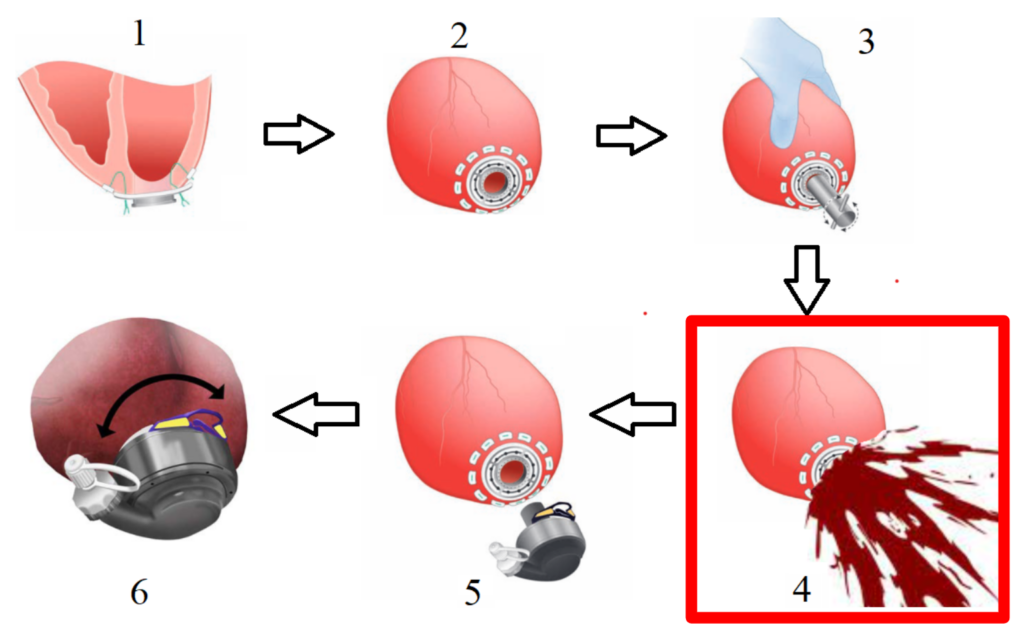
Before inserting the pump, a coring device is advanced through the heart within the apical cuff, creating a hole for the inflow cannula of the pump. This pump directs blood externally to the ascending or descending aorta [3]. Upon coring tool removal, the pressure difference created at the left ventricular apex, coupled with the contractile properties of heart muscle cells (myocytes), causes blood to splash uncontrollably outward. This elevated pressure results from the left ventricle’s thicker wall and higher blood-pumping pressure, facing a substantial workload and mechanical afterload [4].
As previously described, our project aims to develop a mechanism to reduce and potentially control blood splashing. There is no dedicated alternative for addressing blood splashing during LVAD surgery. However, some cardiologists consider employing the cardiopulmonary bypass machine (CPB), designed to temporarily assume the functions of the heart and lungs during surgery. While the primary intent of the CPB is not to mitigate blood splashing, its use has been associated with a reduction in splashing following coring device removal. Nonetheless, complications, including bleeding, cannula malposition, heightened inflammation, and coagulation, pose risks to a patient’s post-surgery health.
BioDesign Statement
A way to perform off-pump LVAD implant to prevent blood splash and eliminate risk of thrombosis for end-stage heart failure patients.
Rationale
Currently, the only technology used to mitigate this problem involves using a cardiopulmonary bypass machine [5]. This bypass device redirects the blood from the heart chambers and reduces the possibility of the splash during coring. It has more risk than reward. It is invasive and leads to embolism along with the additional cost of services and operation of personnel during the surgical procedure [5]. Some surgeons navigate the splash by resorting to making preliminary incisions in the apical region of the heart, reducing left ventricular internal pressure before coring. This method can lead to possible trauma and difficulty in proper coring techniques during the surgical procedure [6].
Two potential methods that can be applied to this problem include the use of the iris diaphragm design and the valves seen in a trocar obturator device [7] [8]. The iris diaphragm is found in the aperture of a camera lens, and the rotatory shutter motion can be useful in closing the apical cuff, but the blades are usually rigid and stand the risk of breaking while interacting with the coring knife during the coring procedure. The trocar obturator is used to initially puncture the abdominal wall, to insert a cannula for subsequent instrument insertion, and a valve mechanism to maintain pneumoperitoneum and prevent gas leakage. The fluid anti-regurgitation control mechanisms in this device offer a lot more promise and can be adapted to suit the specific requirements of our needs.
Requirements
These are the requirements for the SplashGuard device:
- Easy to attach and detach to the apical cuff before and after the coring procedure.
- Remain attached to the apical cuff throughout the coring procedure.
- Prevent the continuous unidirectional flow of blood from the heart to the direction of the attending surgeons immediately after the coring tool is removed.
- Allow the coring knife to go through its center and come out unimpeded.
- Does not cause blood clot formation during its use.
- Lightweight and does not deform the apical cuff.
- Inner core region is transparent.
- Does not release any foreign particles into the LV.
Prototype
Figure 2. SplashGuard prototype in order of: front view, side view, top view (valve compartment), and the iris mechanism (left side = opened, right side = closed) of the prototype.
The SplashGuard comprises 3 parts: the main compartment, the iris mechanism, and the valve compartment, all of which are 3D printed using PLA and tough PLA filament. The main compartment is designed to attach and detach to the apical cuff through a lock and key mechanism and holds 2 lateral venting ports that can be attached with IV tubes to redirect blood. The iris mechanism sits on top of the main compartment, which is the first layer of protection, allows surgeons to freely open and close to control blood flow. The valve compartment serves as the second layer of protection, which is similar to the obturator valves. It is injection molded for flexibility and transparence.
The SplashGuard is designed to be a single use device, with the average use time of 10 minutes. The SplashGuard would be attach after the apical cuff attachment in step 2 as shown in Figure 1. Before proceeding with the coring procedure, surgeons have to make sure the handle is turned to the left so that the iris mechanism is opened and IV tubes are attached to the 2 venting ports. After the coring tool is removed, surgeons would turn the handle to the right to close the iris mechanism and any blood exits the LV would be captured and redirected outside in a peaceful manner by the SplashGuard. Surgeons can see through the transparent valve compartment into the LV to assess the patients’ hearts condition before detaching the SplashGuard and resume the LVAD implant procedure from step 5.
Currently we are in the testing phase, where we are getting promising results that the SplashGuard completely prevents the blood splash and redirects blood in a predictable manner after coring the apex. Through our intellectual property searches, our device is a novel idea without any predicate device. However, Abbott, our main competitor and also possibly going to be a leading customer, could be developing a newer version of their coring tool to accommodate for the blood splash issue. Although we have not seen any patent filing in that space. The SplashGuard is a unique medical device that offers comfort to surgeons and safety to patients. It not only prevents the omnidirectional escape of fluids during the coring process but also reduces the amount of blood loss, streamlines the workflow for surgeons, and reduces the risk of thrombosis for patients. This eliminates the need for the CBP machine which the conventional standard in such procedures despites it having shortcomings such as postoperative embolism and other complication.
Testing
Our testing began with creating a system that mimics the heart during the coring process. We built a testing system with PVC pipes, O-rings, valve, and pressure gauge as seen in Figure 3. The entire system is sealed with PVC glue and is leakproof before testing data is recorded. In addition, the testing system is positioned 45 degrees from the ground to mimic the heart actual position during the coring procedure. The coring tool and the apical cuff is rebuilt in SolidWorks from measurements obtained from the actual HeartMate 3 coring tool and apical given to us by Dr. Gosev and 3D printed using tough PLA.
Splash Reduction Testing
The SplashGuard has successfully demonstrated the capability to capture most of the blood splash during the coring process and after the removal of the coring knife. We have quantified the splash volume with and without the SplashGuard for further analysis, which shows a significant 70% reduction in splash volume as shown in Figure 4.
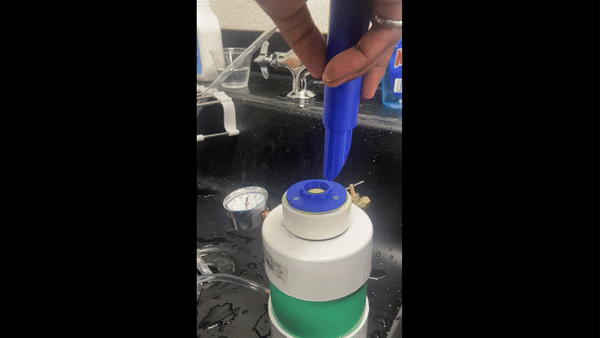
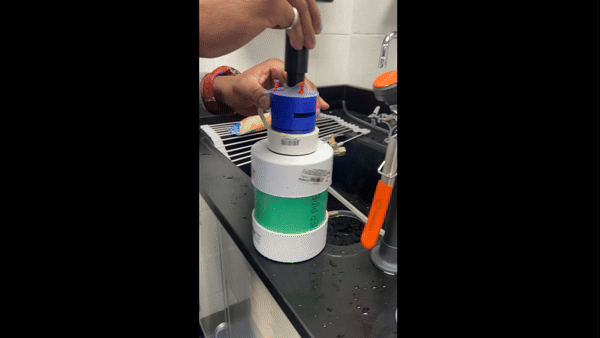
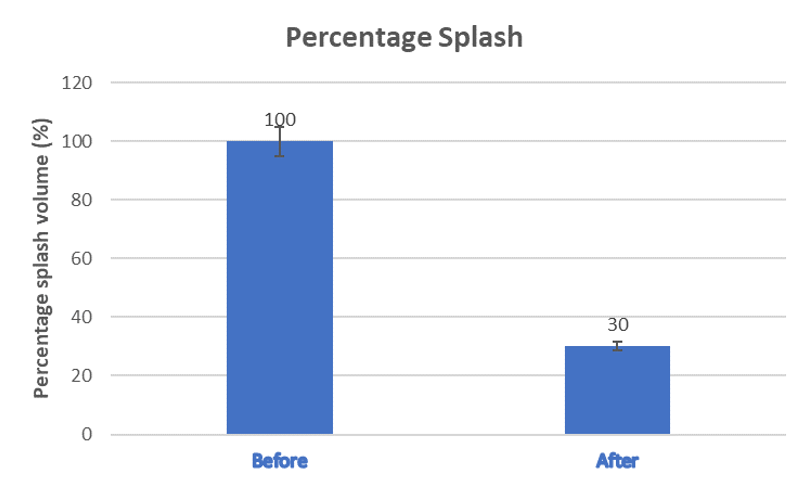
Valve Mechanical Testing
The average LV pressure is 0.007-0.016 MPa. From the Young Modulus and Stress-Strain curve comparison of Ecoflex-20 and Ecoflex-50, Ecoflex-50 would be ideal in handling the escaped pressure of the blood volume during the coring procedure due to its superiority in mechanical strength while maintaining the transparency and obstruction-free to the coring tool.
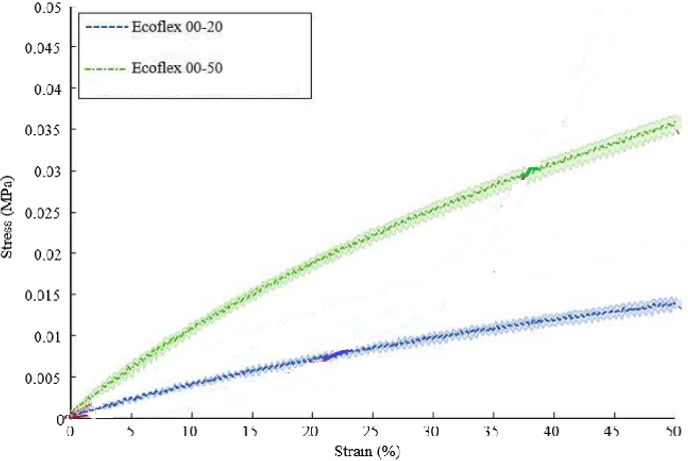
Unit (MPa) | Ecoflex-20 | Ecoflex-50 |
Young Modulus | 1.103 | 2.172 |
Tensile Strength | 0.055 | 0.082 |
Future
Our next step would be continuing our testing and filing a provisional patent to protect our idea since we would be publicizing our idea on Design day. Per our teleconference with the FDA in April 2024 after our PreSub, our future testing should perform the ASTM 2888-13 Standard Test Method for Platelet Leukocyte Count and adhere by ISO 10993-1, “Biological evaluation of medical devices – Part 1: Evaluation and testing within a risk management process” – Guidance for Industry and Food and Drug Administration Staff. Moving forward, we would have a 1 year period to file a non provisional patent for our device and file a Pre Market Approval for the SplashGuard since it is an accessory to a class III medical device (Abbott HeartMate 3). We would then need to prove the SplashGuard can work safely with the HeartMate 3 through rigorous testing before our device is ready for commercialization.
Acknowledgement
We would like to express our deepest appreciation to our customer Dr. Igor Gosev for providing us the opportunity to work on an amazing project and assisting us during the testing phase of the project. We are extremely grateful to our supervisor Dr. Greg Gdowski for guiding us throughout the project, providing us with constructive criticism, and pushing us to deliver our best. Thank you to Martin Gira for providing the team with tools, equipment, and materials throughout the course of the project as well as your knowledge of 3D printing processes. We would like to extend our thanks and appreciation to Dr. Katherine Wood, Dr. Jean-Philippe Couderc, Taimi Marple, and Mahllet Beyene for meeting with us throughout the past two semesters and providing us with helpful resources. Last but not least, we extend our gratitude to our colleagues who have contributed in various ways to make this project successful. Their valuable inputs and support have been invaluable to us.